The Milky Way has always seemed a little bit weird in comparison to other galaxies. For decades, research suggested that our galaxy was more compact than any other spiral galaxy.
In a new study, astronomers reexamined how matter is distributed throughout the Milky Way by tracking the locations and distances of nearly a quarter of a million Red Giants, the enormous, evolved stars that are among the brightest common stars in the galaxy. They found that it’s less strange than we thought—our galaxy’s core is about as dense as any other.
Gail Zasowski, associate professor in the Department of Physics & Astronomy at the U, explained the study that was published on June 27, 2024, in Nature Astronomy.
What made y’all reexamine our Milky Way galaxy?
Our understanding about how galaxies evolve and enrich the universe is driven by models of what is happening inside our galaxy, a sample size of one. For decades, evidence has told us that the Milky Way is a little bit of a weirdo as far as galaxies go—our disk was much more compact than many other spiral galaxies we’ve studied.
Imagine the Milky Way as a vinyl record. The average density of stars, gas, and dark matter is highest in the center of the “record.” As you move towards the edges, everything thins out. Earth is about two thirds of the way towards the outside of the record. We have a good idea about how star density drops off from Earth towards the galaxy’s edge. Previous research estimated the size of the Milky Way by extrapolating the distribution near Earth to star density everywhere in the galaxy.
For the first time, our group used a new data set of Red Giants that were far enough away from Earth to directly measure if our weirdly dense disk was really an outlier. It turns out not to be true.
Why is it hard to measure the Milky Way galaxy?
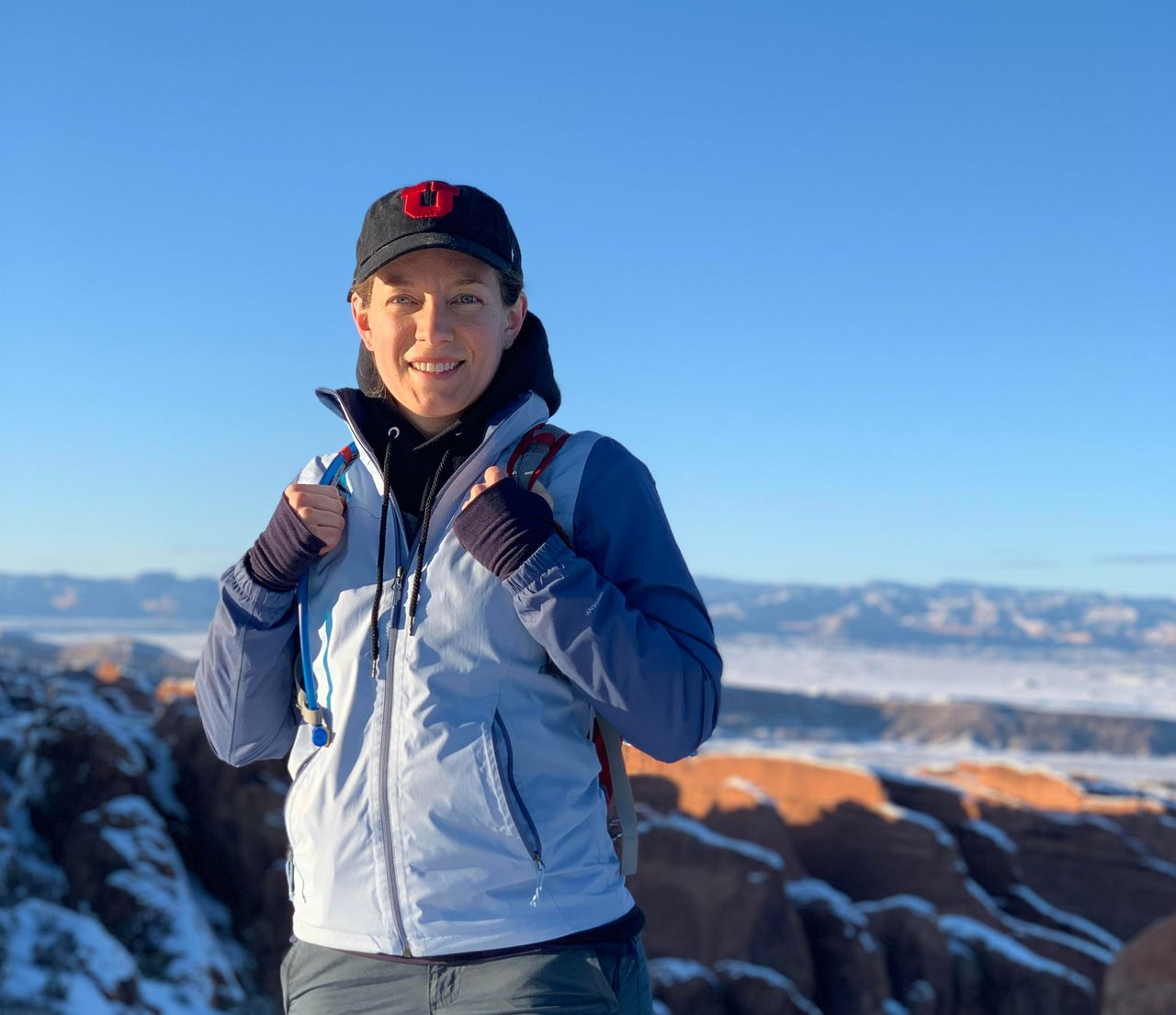
PHOTO CREDIT: Gail Zasowski
Gail Zasowski, associate professor in the Department of Physics & Astronomy at the U.
Download Full-Res ImageIt’s important to understand that the fundamental properties we measure in other systems, like how big they are, how bright they are, what color they are, is exactly the kind of thing we can’t really do in the Milk Way because we’re stuck inside of it.
The perspective we have of our galaxy is akin to standing on a street corner in the middle of downtown Salt Lake City. You can see the shop fronts. You can smell the cars. You can hear the sounds. But you don’t know where the parks and the rivers or even where the mountains are. For external galaxies, the perspective is more like looking at a map of the city where you can see the layout more clearly, but you don’t get a good sense of the finer details. For decades, we’ve assumed that the details gleaned from our Milky Way applied to other galaxies. That’s like mapping Salt Lake City based on the traffic on 100 S.
What new way did you measured the size of the Milky Way?
A common way to measure the size of the Milky Way is called scale length, which asks how quickly the number of stars is dropping off as you move away from the core. In external galaxies, we often use a different measure, called the half-light radius, which is what we did in this paper. Basically, we measure the distance you must go in the galaxy before half of the total light is inside that radius. To do that, you need to be able to see all of the galaxy’s light, then figure out where you have to go to get half of it.
Red Giants are a phase of stellar evolution that many stars go through. There are lots of them in the Milky Way and they’re really, really, bright so you can see them from very far away. The advantage of this data set is that we’re able to use infrared light from the Red Giants that we can’t see with our eyes, but you can see through all the dust floating between us and the stars. Rather than fitting an equation or assuming that there was some shape to the density profile, we were able to directly map out how many stars there are as a function of distance from the center of the galaxy. When we did that, we got a number that is very much in line with other systems that we think are the same mass and other similar global properties to ours.
Did anything surprise you?
My research focus is the chemical evolution of galaxies, or how many heavy elements the stars have in them. This goes back to the fact that virtually every heavy element on the periodic table is made during the life and death of a star. We think that in most galaxies, the earliest stars started forming in the very center, so they started making heavy elements earlier. You form stars later and later as you move outwards from the center. Those tend to have lower amounts of heavy elements in there. Tracking how heavy elements drop off in a galaxy can tell you things like how rapid the star formation was at different times in its history, how many of those heavy elements did it throw out as gas between galaxies never to be seen again?
This is where the Milky Way might still be a weirdo. The element gradient made sense in the context of the Milky Way’s previous size. But now, that gradient of heavy elements is different. So, the Milky Way may be more normal in terms of its physical size for its mass. But it may be weird in other ways.
Study credits:
The paper is the result of the Apache Point Observatory Galactic Evolution Experiment (APOGEE), a survey that uses data from the Sloan Telescope at the Apache Point Observatory and the du Pont Telescope at Las Campanas Observatory in Chile.
APOGEE is part of the Sloan Digital Sky Survey, one of the most highly cited, most impactful surveys in the world of which the University of Utah has been a part of decades.
All data from the SDSS is stored at the U’s Center for High Performance Computing and is fully public.
Other authors of the paper include: Jianhui Lian, Bingqiu Chen, Tao Wang, and Xiaowei Liu of Yunnan University; Julie Imig of the Space Telescope Science Institute, and Nicholas Boardman of the University of St. Andrews.
MEDIA & PR CONTACTS
-
Lisa Potter
Research communications specialist, University of Utah Communications
949-533-7899 lisa.potter@utah.edu