Astronomy’s new blockbuster was announced in New Orleans during the 2024 American Astronomical Society meeting. Astronomers from the Sloan Digital Sky Survey (SDSS) discovered evidence for what they call the “Barbenheimer Star”—an enormous ancient star that exploded in a way previously thought impossible, resulting in an unusual pattern of elemental ashes that left behind a trail of evidence still visible billions of years later.
“We’ve never seen anything like this,” says Alex Ji of the University of Chicago and SDSS, the lead author of the study. “Whatever happened back then, it must have been amazing. We nicknamed it the ‘Barbenheimer Star’ for its spectacular nucleosynthesis.”
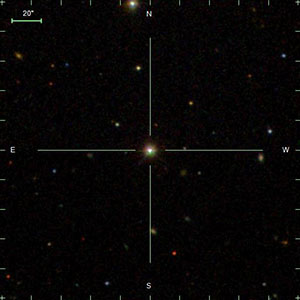
PHOTO CREDIT: Jordan Raddick and the SDSS-V collaboration
The star J0931+0038, as seen by the Sloan Digital Sky Survey camera in 1999.
Download Full-Res ImageJi and colleagues didn’t see the Barbenheimer Star directly. Instead, they followed the trail back in time using a process called “stellar archaeology.” Just as archaeologists use evidence found in the present to reconstruct the past, astronomers use evidence found in today’s stars to reconstruct conditions in the ancient universe. Today’s stars are like chemical time capsules—they preserve what a piece of the universe was like when the star was born.
“As we continue to map the sky, obtaining millions of spectra across the galaxy and extra-galactic black holes, astronomers are making great strides in adding to our understanding of how objects in the universe evolve,” said Joel Brownstein, research associate professor in the University of Utah’s Department of Physics & Astronomy and co-author of the study.
Brownstein is the head of data for SDSS and runs the Science Archive Server (SAS), which is hosted by the U’s Center for High Performance Computing. The SAS stores data transferred to Utah from the survey’s telescopes at Apache Point Observatory in North America and Las Campanas Observatory in South America. To manage the massive data flow, Brownstein led the effort to manage the pipelines that run on the SAS, which perform the scientific data reductions for shepherding the raw data from the telescopes into usable information, known as spectra, for thousands of SDSS members to access and analyze.
“It’s like making a daily feast,” Brownstein said. “Only a few people might make the meal’s courses, but everyone sits down to dinner. The pipelines are cooked by a few people, but millions of individual spectra and their associated parameters are consumed by thousands of people in the collaboration.”
The many collaborators can access the raw data at any time. Ji, the Barbenheimer Star paper’s lead author, initiated the analysis through the SAS platform.
“We’re all very closely working together. None of that would have been possible without Utah, without this central place where we can share ideas and look at each other’s output,” Brownstein said.
The trail of evidence began with a star that, at first glance, appears unremarkable. The star, called J0931+0038, is a distant, bright red star captured in an SDSS image way back in 1999. Twenty years later, the SDSS telescope turned once again to the star – this time in technicolor. The SDSS Milky Way Mapper program observed the star’s spectrum, which measures how much light the star gives off at different wavelengths. A spectrum can reveal many things about a star, such as its temperature and chemical composition—and it was chemistry that first led Ji and his team of stellar archaeologists to notice J0931+0038.
Stars are mostly made of hydrogen and helium, but they also incorporate some of the heavier elements, which were created in previous generations of stars and released into the universe in supernova explosions. These heavier elements show up as prominent valleys in a star’s spectrum. The SDSS spectrum indicated that J0931+0038 had an unusually low amount of magnesium, prompting further follow up from the Magellan telescopes in Chile. When Ji and colleagues first viewed the follow up spectrum of J0931+0038, they were amazed.
“As soon as I saw the spectrum, I immediately emailed the rest of the team to talk about how to learn more,” Ji said.
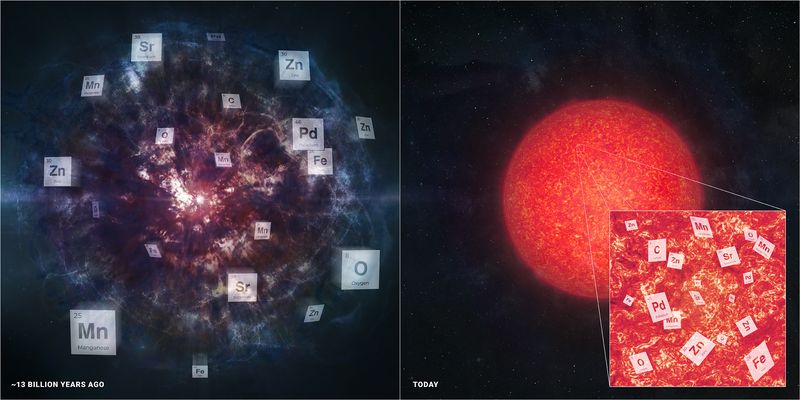
PHOTO CREDIT: University of Chicago/SDSS-V/Melissa Weiss
(Left) Long ago, the supernova explosion of the Barbenheimer Star releases an unusual mix of chemical elements in to nearby gas clouds. (Right) Today, we can look at J0931+0038 to see that unusual mix of elements and reconstruct the history of the Barbenheimer Star.
Download Full-Res ImageSeveral things made the star different from other stars: low abundances of elements with odd numbers on the periodic table like sodium and aluminum; a large amount of elements close to iron in the periodic table like nickel and zinc; and an overabundance of heavier elements like strontium and palladium.
“We sometimes see one of these features at a time, but we’ve never before seen all of them in the same star,” says Jennifer Johnson of the Ohio State University, another member of the stellar archaeology team.
So what made J0931+0038 look the way it looks? The star formed from the supernova remnant of whatever star was there before – and so its unusual composition means that the star that was there before must have also been highly unusual. It is that ancient, weird star whose remains we see preserved today that astronomers have nicknamed the “Barbenheimer Star.”
Which elements are left behind after a supernova explosion depend on the mass and chemical composition of the exploded star, and also on the details of how it exploded. Whatever the Barbenheimer Star was, it must have been a blockbuster—at least 50 to 80 times the mass of our sun. In fact, that ancient supernova must have been so massive that astronomers are surprised it could happen at all; previous theories predicted that such big stars should collapse straight into black holes, without creating a supernova first. As surprising as it is to learn that such a massive star could go supernova, even that doesn’t explain the full picture.
“Amazingly, no existing model of element formation can explain what we see,” says Sanjana Curtis of the University of California, Berkeley, co-lead of the published study. “It’s not just, ‘oh, you can tweak something here and there and it’ll work out’—the whole pattern of elements looks almost seems self-contradictory.”
The best way to resolve the apparent contradiction is for astronomers to take two approaches simultaneously. First, we need more and better computer simulations to make predictions about what happened with stars in the early universe to create the stars we see today. Second, we need more observations of today’s universe to provide evidence to evaluate the computer simulations. Considering that the SDSS team discovered evidence for the Barbenheimer Star the very first night they followed up their initial observations, we can expect many more blockbuster results in the coming years.
“The universe is our laboratory, but we don’t have direct access to tweak the parameters or change our perspective or rerun certain events,” said Gail Zasowski, associate professor of physics and astronomy at the U and former spokesperson of SDSS. “We have to work with the ancient signatures and fossils that we can find, to recreate what came before.”
About the Sloan Digital Sky Survey
Funding for the Sloan Digital Sky Survey V has been provided by the Alfred P. Sloan Foundation, the Heising-Simons Foundation, the National Science Foundation, and the Participating Institutions. SDSS acknowledges support and resources from the Center for High-Performance Computing at the University of Utah. SDSS telescopes are located at Apache Point Observatory, funded by the Astrophysical Research Consortium and operated by New Mexico State University, and at Las Campanas Observatory, operated by the Carnegie Institution for Science.
SDSS is managed by the Astrophysical Research Consortium for the Participating Institutions of the SDSS Collaboration, including Caltech, the Carnegie Institution for Science, Chilean National Time Allocation Committee (CNTAC) ratified researchers, The Flatiron Institute, the Gotham Participation Group, Harvard University, Heidelberg University, The Johns Hopkins University, L’Ecole polytechnique fédérale de Lausanne (EPFL), Leibniz-Institut für Astrophysik Potsdam (AIP), Max-Planck-Institut für Astronomie (MPIA Heidelberg), Max-Planck-Institut für Extraterrestrische Physik (MPE), Nanjing University, National Astronomical Observatories of China (NAOC), New Mexico State University, The Ohio State University, Pennsylvania State University, Smithsonian Astrophysical Observatory, Space Telescope Science Institute (STScI), the Stellar Astrophysics Participation Group, Universidad Nacional Autónoma de México, University of Arizona, University of Colorado Boulder, University of Illinois at Urbana-Champaign, University of Toronto, University of Utah, University of Virginia, Yale University, and Yunnan University.
MEDIA & PR CONTACTS
-
Lisa Potter
Research communications specialist, University of Utah Communications
949-533-7899 lisa.potter@utah.edu