Traditional electronics use semiconductors to transmit data through bursts of charged carriers (electrons or holes) to convey messages in “1s” and “0s.” Spintronic devices can process an order of magnitude more information by assigning binary code to the orientation of electrons’ magnetic poles, a property known as spin— an “up” spin is a 1, a “down” is a 0.
A major barrier to commercial spintronics is setting and maintaining the electron spin orientation. Most devices tune spin-orientation using ferromagnets and magnetic fields, a burdensome and unreliable process. Decades of research has shown that carriers lose their spin orientation moving from materials with high-conductivity to low-conductivity—for example, from metallic ferromagnets to undoped silicon and conjugated polymer materials that make up most modern semiconductors.
For the first time, scientists transformed existing optoelectronic devices into ones that can control electron spin at room temperature, without a ferromagnet or magnetic field.
Most optoelectronic devices, such as LEDs, only control charge and light but not the spin of the electrons. In the new study led by the University of Utah physicists and researchers at the National Renewable Energy Laboratory (NREL), replaced the electrodes of store-bought LEDs with a patented spin filter, made from hybrid organic-inorganic halide perovskite material. The LEDs produced circularly polarized light, a tell-tale sign that the filter had injected spin-aligned electrons into LED’s existing semiconductor infrastructure, a massive step forward for spintronics technology.
“It’s a miracle. For decades, we’ve been unable to efficiently inject spin-aligned electrons into semiconductors because of the mismatch of metallic ferromagnets and non-magnetic semiconductors,” said Valy Vardeny, Distinguished Professor in the Department of Physics & Astronomy at the U and co-author of the paper. “All kinds of devices that use spin and optoelectronics, like spin-LEDs or magnetic memory, will be thrilled by this discovery.”
The study was published in the journal Nature on June 19, 2024.
Spin filters
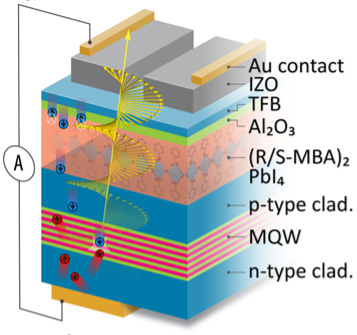
PHOTO CREDIT: Hautzinger, M. et al. Nature (2024)
Stack of the spin-LED emitting circularly polarized electroluminescence. The (R-MBA2Pbl) acts as a spin filter, allowing only polarized carriers (blue circles) to flow through the LED and recombine in the multiple quantum wells (MQW)s emitting circularly polarized light (yellow helix).
Download Full-Res ImageIn 2021, the same collaborators developed the technology that acts as an active spin filter made of two successive layers of material, called chiral hybrid organic-inorganic halide perovskites. Chirality describes molecule’s symmetry, where its mirror image cannot be superimposed on itself. Human hands are the classic example; hold yours out, palms facing away. The right and left hands are arranged as mirrors of one another—you can flip your right hand 180° to match the silhouette, but now the right palm is facing you while the left palm faces away. They’re not the same.
Some molecules, such as DNA, sugar and layers of chiral hybrid organic-halide perovskites, have their atoms arranged in chiral symmetry. The filter works by using a “left-handed” oriented chiral layer to allow electrons with “up” spins to pass, but block electrons with “down” spins, and vice versa. At the time, the scientists claimed the discovery could be used to transform conventional optoelectronics into spintronic devices simply by incorporating the chiral spin filter. The new study did just that.
“We took an LED from the shelf. We removed one electrode and put the spin filter material and another regular electrode. And voila! The light was highly circularly polarized,” said Vardeny.
Chemists from the NERL fabricated the spin LEDs by stacking several layers, each with specific physical properties.The first layer is a common transparent metallic electrode; the second layer’s material blocks electrons having spin in the wrong direction, a layer that the authors call a chirality-induced spin filter. The spin-aligned electrons then recombine in the third layer, a standard semiconductor used as an active layer in regular LEDs. The injected spin aligned electrons cause this layer to produce photons that move in unison along a spiral path, rather than a conventional wave pattern, to produce the LED’s signature circular polarized electroluminescence,
“This work demonstrates the unique and powerful ability for these emerging ‘hybrid’ semiconductors to combine and take advantage of the interplay of the distinct properties of organic and inorganic systems,” said Matthew Beard, coauthor of the study of NREL. “Here the chirality is borrowed from the organic molecules and provides control over spin while the inorganic component both orients the organic component and provides conductivity or control over charge.”
Once they installed the filter into a standard LED, Xin Pan, research assistant in the Department of Physics & Astronomy at the U, confirmed that the device worked as intended, namely by spin-aligned electrons. However, more research is needed to explain the exact mechanisms at work to create the polarized spins.
“That’s the $64,000 question for a theorist to answer,” said Vardeny. “It’s really a miracle. And the miracle is without knowing the exact underlying mechanism. So that’s the beauty of being experimentalist. You just try it.”
The authors assert that other scientists can apply the technique using other chiral materials, such as DNA, in many contexts.
*******
The study, “Room-temperature spin injection across a chiral perovskite/III-V interface,” was published in Nature on June 19, 2024.
Other authors include Xin Pan from the University of Utah; Matthew Hautinger, Steven Hayden, Qi Jiang Mickey Wilson, Alan Phillips, Yifan Dong, Emily Raulerson, Ian Leahy, Chun-Sheng Jian, Jeffrey Blackburn, and Katherine Jungjohann from the National Renewable Energy Laboratory (NREL); Jiselle Ye and Alan Phillips from NREL and the Colorado School of Mines; Joseph Luther, Kirstin Alberi and Joseph Berry from NREL and the University of Colorado Boulder (UCB); and Yuan Lu from Université de Lorraine. The work was supported as part of the Center for Hybrid Organic Inorganic Semiconductors for Energy (CHOISE) Energy Frontier Research Center in the U.S. Department of Energy, and the French National Research Agency.
MEDIA & PR CONTACTS
-
Lisa Potter
Research communications specialist, University of Utah Communications
949-533-7899 lisa.potter@utah.edu