On June 28, dozens of astronomy enthusiasts gathered on the University of Utah campus to watch a live stream of a mysterious announcement. For weeks prior, scientists on Twitter, TikTok and IRL were abuzz with anticipation, awaiting results from the North American Nanohertz Observatory for Gravitational Waves’ (NANOGrav) 15 years’ worth of data. NANOGrav confirmed what had long been suspected—gravitational waves are thrumming throughout the universe, emitting a low-pitched symphony that distorts the fabric of space and time.
Tanmoy Laskar, assistant professor at the U’s Department of Physics & Astronomy thought of organizing the watch party to share in the excitement and discuss the results with the U community. He spoke with @TheU to explain the announcement.
Why is the astrophysics community so excited about the announcement?
This is very exciting because our current astrophysics and cosmology theories tell us that the universe should be full of these gravitational waves and, with these new results, the evidence for the existence of such a gravitational wave background just got much, much stronger. Furthermore, multiple global teams published their own, independent data sets on the same day and each team finds strong evidence for the presence of this gravitational wave background, which means that this signal is very likely real.
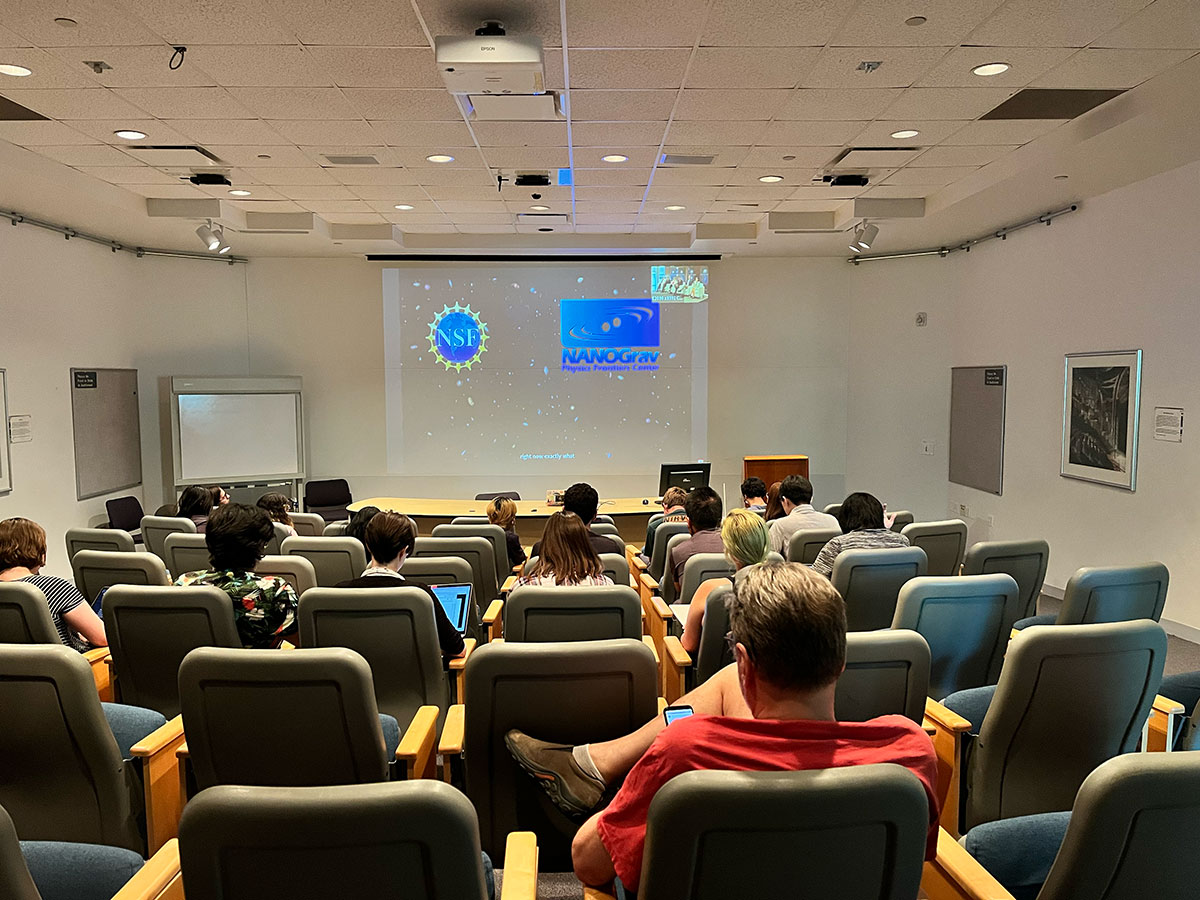
PHOTO CREDIT: Tanmoy Laskar
A live stream watch party for the NANOGrav announcement at the U.
Download Full-Res ImageHow did the collaboration detect the gravitational waves?
Gravitational waves are essentially a small stretch and squeeze in space and time. This means that if we want to detect a gravitational wave going by, we need to measure small perturbations to the distance between free-floating masses or to the time difference between two freely falling clocks. But the gravitational wave background that was the focus of the new studies involves waves with extremely long wavelengths—dozens of light years. This means to detect them we need clocks or masses separated by similar distances.
To navigate this, NANOGrav and their sister experiments used a technique called pulsar timing. Pulsars are rapidly spinning, very dense stars packing the mass of our sun into the size of a small city. They were discovered in 1967 by Dame Jocelyn Bell-Burnell as extraterrestrial objects producing regular radio pulses. The radio pulses from pulsars tend to be extremely regular because they behave similar to lighthouses. If you look at a lighthouse from the shore, its rotating beam of light flashes towards you at regular intervals. In the same way, pulsars appear as regular radio pulses seen when their lighthouse-like radio beams periodically sweep past Earth.
Astronomers realized that an array of pulsars spread across our galaxy could be used as a network of clocks. By timing the arrival of the pulses from these pulsars, one could look out for passing gravitational waves that would disrupt the timing of radio pulses that would usually arrive like clockwork. Tracking a large number of pulsars for disruptions is much more reliable. The idea is that if a gravitational wave goes through, then not only will there will be offsets in the time of arrival of the pulses from each pulsar from their expected times, such effects will be correlated in a predictable fashion between different pulsars depending on each pulsar’s direction and distance from Earth.
Of course a lot of different effects still need to be accounted for, including the motion of the Earth and planets in the Solar System and the slowing down of each pulsar as it slowly loses energy. Not to mention the fact that these gravitational waves have wavelengths that correspond to several Earth years, meaning that the observations need to be collected for over a decade to make a discovery!
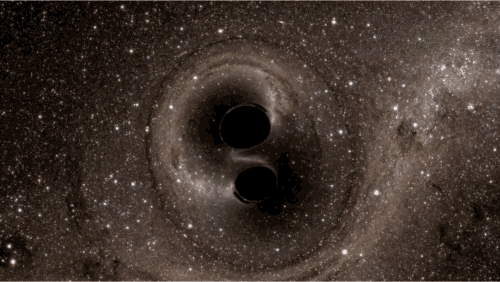
PHOTO CREDIT: SXS via NASA
This computer simulation shows the collision of two black holes, which produces gravitational waves.
Download Full-Res ImageWhat caused the gravitational-wave signals?
The origin of the gravitational wave is not definitive, partly because the pulsar array technique is indirect, and partly because the data do not yet reach the sensitivity to be able to resolve individual sources. The observed signal appears to be consistent with theoretical expectations of gravitational waves produced by binary systems comprising supermassive black holes, although more exotic scenarios can’t be definitively ruled out yet.
I’ve read that the discovery is in line with Einstein’s theory of general relativity—how so?
We can estimate how many binary black holes we expect to exist in the universe based on our current understanding of how galaxy’s form and grow. Knowing this, we can use Einstein’s theory of general relativity to calculate how “loud” the gravitational wave background should be. The NANOGrav signal is consistent with that expectation, so indirectly this is yet another test of the theory of general relativity.
Does this discovery impact how you think about your research focus?
I study individual sources of gravitational waves that also emit light, in particular, merging neutron stars. Learning about gravitational waves from different astrophysical sources and with different techniques helps us develop a better understanding of how gravitational waves are produced. It also gives us more opportunities to use gravitational waves for testing fundamental physics like Einstein’s theory of relativity at many different scales of size and energy.
How does this change what we know about the universe?
The gravitational wave background is to a pulsar timing array system a bit like going to a party, where there’s lots of background chatter, but we can’t quite tell what any one individual is saying. As astronomers collect more timing data over the years, we might be able to start resolving individual sources of gravitational waves, which would be like picking out individual conversations in the party. The successors to the current generation of pulsar timing array experiments might also be able to pick out the very faint hum of gravitational waves expected to have been produced when the universe was born, that still dance around the universe today — that would be yet another amazing discovery!
MEDIA & PR CONTACTS
-
Lisa Potter
Research communications specialist, University of Utah Communications
949-533-7899 lisa.potter@utah.edu