Research led by University of Utah chemists has resulted in new composite materials capable of converting long low-energy lightwaves to higher-energy light, potentially opening more efficient avenues for transferring energy.
According to a recently published study, Ming Lee Tang and colleagues developed a method for joining minute silicon crystals with organic carbon-based molecules to develop hybrid compounds with optoelectronic properties that could enhance numerous technologies that harness light.
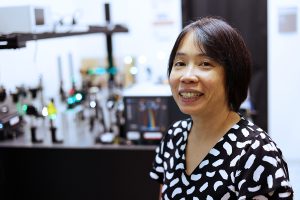
PHOTO CREDIT: Todd Anderson
U chemistry professor Ming Lee Tang
The discovery hinges on the strong chemical bonds her lab was able to achieve between two completely different materials, silicon and hydrocarbons.
“We use those excited states that are not the same as in the bulk materials in your computer,” said Tang, an associate professor of chemistry who came to the U from the University of California Riverside two years ago. “They absorb more strongly and you can also convert the energy in different ways that are not allowed in the bulk material. It’s different physics.”
The lab, known as the Tang Group, is funded by the U.S. Department of Energy, Air Force and National Science Foundation to develop novel energy conversion processes involving inorganic nanoparticles and organic molecules. The team’s mission is “to find synergistic combinations of conjugated molecules and nanoparticles where the sum is more than its parts,” according to its website. “The goal is to enhance electronic communication between quantum-confined inorganic nanomaterials and organic compounds.”
The lab’s latest findings were reported last month in the journal Nature Chemistry.
“This process gives us a whole new way of designing materials,” said co-author Sean Roberts in a news release posted by the University of Texas. “It allows us to take two extremely different substances, silicon and organic molecules, and bond them strongly enough to create not just a mixture, but an entirely new hybrid material with properties that are completely distinct from each of the two components.”
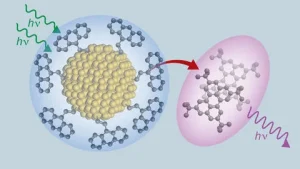
A new class of composite materials can turn long-wavelength photons into short-wavelength blue or ultraviolet photons. (University of Texas, Austin)
Properties associated with these novel materials, which can “upconvert” long-wavelength photons in red light to shorter wavelengths in blue and violet light, could prove useful in various light-driven applications, including bioimaging, 3-D printing, photovoltaics and sensors, according to Roberts, a chemistry professor at the Austin campus.
Central to the research are silicon nanoparticles, or quantum dots, that Tang’s team fabricates in their U lab. These infinitesimal crystals measure about 5 nanometers, so tiny that they behave differently than silicon in a “bulk” state. (The width of a human hair is about 16,000 times larger than these nanocrystals.)
Tang hypothesized that silicon nanoparticles could form strong covalent bonds with carbon atoms where electrons are equally shared. Such bonds could allow for greater electronic coupling between the crystals and the organic molecules attached to their facets.
The second most abundant element in Earth’s crust, silicon is rare in a pure form. At No. 14 on the Periodic Table, silicon is stacked between carbon and germanium in a column of tetravalent elements, meaning their outer rings hold four electrons.
Because it is a good semiconducting material, meaning it both conducts and blocks electrical currents, silicone has countless applications in electronics, including photovoltaics. One drawback, however, this crystalline metal absorbs light only at the lower reddish end of the electromagnetic spectrum.
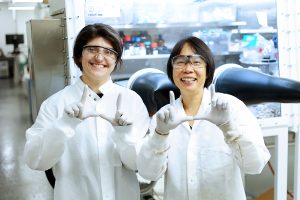
PHOTO CREDIT: Todd Anderson
U chemist Ming Lee Tang, right, and her graduate student Maryam Baraazandeh investigate ways to make hybrid materials with unique optoelectronic properties.
But the light silicone can absorb is stuck at the redder end of the visible spectrum, where the wavelengths are longer and pack less energy.
After years of work, Tang and her colleagues have now shown they join silicon with a hydrocarbon known as anthracene in ways that increases the speed the two molecules exchange energy.
The resulting strong chemical bond greatly enhances the silicon’s efficiency in converting lower-energy light to higher-energy light.
One application where Tang believes this new material holds great promise is in optogenetics, which is driving experimental neurological research using mice.
“This technology can turn on certain neurons using light. ‘Opto’ because involves light, ‘genetics’ because you can encode it in a neuron,” Tang said. “The problem is that you want to shine blue light or green light and that doesn’t go very far in the brain, even for a mouse brain which is 5 millimeters.”
Tang’s discovery can improve the optical probes researchers implant in the mice’s brains.
“If you can use this upconversion in vivo, you would have a much more realistic experiment with the animal closer to its native environment,” Tang said. “Human brains are too thick for this to happen, but in a mouse brain, it might be viable.
Her research collaboration included the U’s Kefu Wang; R. Peyton Cline and Joel D. Eaves of the University of Colorado Boulder; Joseph Schwan and Lorenzo Mangolini of the University of California Riverside; and Jacob M. Strain of the University of Texas.
The full paper, titled “Efficient photon upconversion enabled by strong coupling between silicon quantum dots and anthracene,” can be found here.
MEDIA & PR CONTACTS
-
Brian Maffly
Science writer, University of Utah Communications
801-573-2382 brian.maffly@utah.edu