Reposted from College of Science.
Why do the vast majority of cancer cases arise late in patients’ lives?
A traditional explanation in the development of cancers is a paradigm focused on mutations in individual cells. According to this model, known as somatic theory, a cascade of approximately six mutational changes in a single cell triggers cancer. This theory explains the rapidly increasing “power function” that describes how cancer incidence rises with a person’s age.
But this power function which lines up with cancer’s six classic hallmarks is now being challenged by a different paradigm that casts doubt on the primacy of individual cells in cancer development. It also challenges the notion that cancer marks a strict change between “normal” and aberrant tissues, particularly as the body ages.
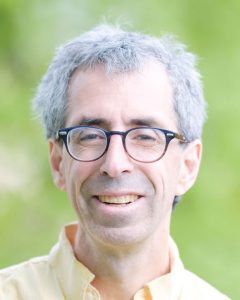
Fred Adler, U mathematics professor and director of the School of Biological Sciences
In a new paper published The Royal Society Interface, University of Utah mathematics professor Frederick Adler explores “a modeling framework for cancer ecology and evolution.”
Adler has struggled for a long time to come up with an alternative modeling approach for cancer that has the flexibility to capture the complexity of cancer, while standing by the dictum that cancer cells are still cells.
“It involved a plane trip where I worked out an extremely complicated approximate version of the method before figuring out, on solid ground, that the exact version was thoroughly simple,” he said.
Simple didn’t just mean elegant, but also getting results in a reasonable amount of time by optimizing code, something he can appreciate as the director of the U’s School of Biological Sciences.
Adler’s findings build on those of others that countermand the primacy of individual cells. These include observations of mutations common in non-cancerous tissues, and sometimes more common than in nearby cancers.
“This implies,” the paper states, “that cancers depend on interactions with the surrounding tissue.” A second emphasis on cancer ecology and evolution is now highlighting “the ecology of nutrients, acids and physical factors and the role of cell interactions.”
“Detailed study of adults shows that few if any of their cells are ‘normal,’” Adler said. “Tissues are instead made up of lineages with ever-increasing numbers of aberrant traits, many of which promote excess growth. The vast majority of these incipient growths are contained by controls within those cells and by other cells.”
In Adler’s parsing of the ecological paradigm, senescence theory plays a critical role, focusing on the breakdown of the system of controls within and around individual cells.
“Many cancers [for example], “develop much later than their originating oncogenic mutations.” Furthermore, mutant cells in his models are restrained “by systems that remove their growth advantage, but which can weaken with age due to changes such as impaired intercellular communication. Remarkable data on genetic diversity in healthy tissues show that cancer-related mutations are ubiquitous, and often under positive selection despite not being associated with progression to cancer.”
Tracking the dual nature of cells
In the paper, Adler first presents a modeling framework which incorporates evolution, stochasticity (a measure of how random a process is, or the quality of lacking a predictable order or plan) and control and breakdown of control. Using a differential equation, the model then tracks the dual nature of individual cells as ecological competitors for resources and space.
Adler then tested whether the ecological model of cancer initiation generates realistic age-incidence patterns similar to the somatic mutation theory. Another test was made to determine how initial defects in the control systems accelerate the process. In this comprehensive systems view, cancer, and an incipient cancer in particular, is not an invader.
“It is a set of cells,” the paper reads, “that escape the many layers of internal and tissue level regulation, and then grow to damage the host. The success of a cancer, or equivalently the failure of the regulatory system, requires that the cancer co-opts or evades the systems of control and repair.”
This model/framework, according to the author, assumes a particular structure of the control system but has capacity for “several other extensions” to make it more “realistic.” Those extensions would address, for example, cell differentiation and a clearer class of driver mutations for the genetic model of quantitative trait. Another might address why the mutualist cells in the tests maintain a constant phenotype in spite of what we know about how cells alter behavior in cancer’s presence.
Statistically, we understand that cancer emerges more frequently in older individuals, but how and why are what Adler is attempting to determine. His model, he said, “reproduces the rapid increase of cancer incidence with age, identifies the key aspects of control, and provides a complement to the focus on mutations that could lead to new treatment strategies.”
Fred Adler points out that the control system in the model differs greatly across species in concert with their body size and lifespan, thus revealing a paradox known as Peto’s: cancer rates are similar across organisms with a wide range of sizes and lifespan.
“This robustness,” concludes the paper, “is a special case of the principle that all biological systems must be overbuilt to deal with uncertainty.” Referencing Shakespeare’s “Hamlet,” Adler states that this development in excess of demand exists “to survive ‘the thousand natural shocks that flesh is heir to’… This model seeks to place those shocks in the ecological and evolutionary context that makes long life possible.”
Read about Fred Adler’s related work in modeling cancer development, specifically with breast cancer.