Adapted from a story posted Dec. 20 by the National Institute of Biomedical Imaging and Bioengineering.
While ultrasound has been widely used for medical imaging, it also has a variety of therapeutic applications. The technology could potentially facilitate the release of medication in precise locations for disorders and conditions that require drug treatment.
Ideally, a drug would be released only in specific regions of interest and at a high concentration, to maximize the benefits and minimize side effects. However, releasing a drug selectively in specific locations in the body, including the brain, has been challenging. Researchers have tackled the problem by designing ultrasound-sensitive nanoparticles that release a drug at the targeted site when activated by focused ultrasound.
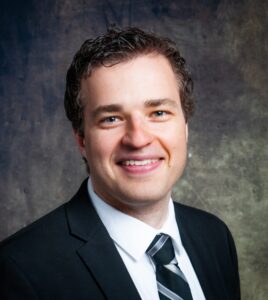
Jan Kubanek
In a proof-of-concept study, University of Utah researchers tested whether this method could release a drug in a specific area of the brain of non-human primates. The results, published in the Journal of Controlled Release, showed that the ultrasound-sensitive nanoparticles released a substantial dose of the anesthetic propofol in specific deep brain regions. The treatment was found to be safe and effective, and the result was reversible.
“The main benefit of using ultrasound-sensitive nanoparticles is that they encapsulate the drug so that it has minimal interaction with the body, except where it’s released by focused ultrasound,” said Jan Kubanek, assistant professor of biomedical engineering and study’s corresponding author. “This could potentially allow us to treat under-regulated or malfunctioning circuits in the brain without exposing the entire brain and the body to drugs.”
Designing nanocarriers to deliver a drug payload
With funding from the National Institutes of Health, the researchers engineered new nanoparticles with three layers: an inner core made up of a contrast agent that responds to ultrasound activation, a second layer that encapsulates the drug, and an outer shell.
The design builds upon previous research in rodents that showed that nanoparticles can contain contrast agents that change from a liquid to gas upon interaction with high-frequency ultrasound waves. This approach facilitates a controlled release of the drug at a precise location.
However, a limitation of previous research was that the nanoparticles were unstable when they entered the bloodstream, which raised safety concerns. Kubanek’s team increased the stability of the nanoparticles by choosing a different contrast agent and adding an outer shell to the design. They also encapsulated the drug to prevent it from interacting with surrounding tissues and organs until it was activated by the ultrasound and released in the targeted brain region.
Evaluation in large animal models
The researchers loaded the nanocarriers with a low dose of propofol, an anesthetic that suppresses neural circuits, to evaluate the safety and effectiveness of their combined method. They used an established visual-choice experiment to determine whether releasing propofol in specific visual brain regions would impact the monkeys’ behavior. Briefly, the animals are shown two targets—a flash of light on the left and right. They indicate which target appeared first by making an eye movement in that direction.
The researchers focused on the right and left lateral geniculate nuclei, or LGN, which are small structures in the brain that are important for vision. Because each LGN derives input from the opposite side, the researchers expected that inhibiting the LGN on one side would affect vision on the other side. For example, administering propofol directly to the right LGN would impair the animal’s visual perception on the left side, and the animal would be biased to choose the targets on the right side.
After propofol-loaded nanoparticles were administered into the bloodstream by injection, the researchers delivered 1-minute ultrasound pulses to the right or left LGN and observed how the animals reacted to the stimuli. As predicted, the animals chose the target on the right when the propofol was released in the right LGN.
The researchers concluded that the selective release of propofol modulated the subjects’ visual choice behavior, which was specific to propofol and the targeted side of the brain when compared with ultrasound alone. The researchers also found that a low dose of propofol achieved a targeted brain delivery when activated by ultrasound and minimized its interaction with tissues and organs. This could indicate that lower doses of drugs could potentially achieve the desired effect.
In addition, the study found that the average time the nanoparticles circulated in the blood was about 30 minutes, which provides a practical time window for applications in humans.
“This study is important because it demonstrated a safe and effective approach to releasing drugs on demand in awake, behaving primates, as opposed to previous studies that used rodents, thus providing a critical step toward future clinical translation,” said Guoying Liu, director of National Institute of Biomedical Imaging and Bioengineering’s Division of Applied Science and Technology.
The Utah researchers aim to eventually apply their formulation to a variety of medications that produce side effects, including chemotherapy.
“A major strength of this approach is that the nanoparticles are designed to carry any drug and release it upon ultrasound activation so this system could be used to treat cancer, pain, or addiction,” Kubanek said. Besides additional testing in non-human primates, the research team is also testing the potential of this targeted release approach in delivering chemotherapy in a mouse model of glioblastoma, the most common, aggressive and deadly brain cancer.
####
The study, “Remotely controlled drug release in deep brain regions of non-and human primates,” was published in the May 2024 edition of the Journal of Controlled Release. Funding for the research came from the National Institute of Biomedical Imaging and Bioengineering, the National Institute of Neurological Disorders and Stroke and the National Institute of Mental Health. Co authors include Matthew Wilson and Taylor Webb of the U’s Department of Biomedical Engineering and Henrik Odéen of the Department of Radiology and Imaging Sciences.
MEDIA & PR CONTACTS
-
Brian Maffly
Science writer, University of Utah Communications
801-573-2382 brian.maffly@utah.edu